Potential for SMA Therapies in Stem Cells for Motor Neuron Acquisition
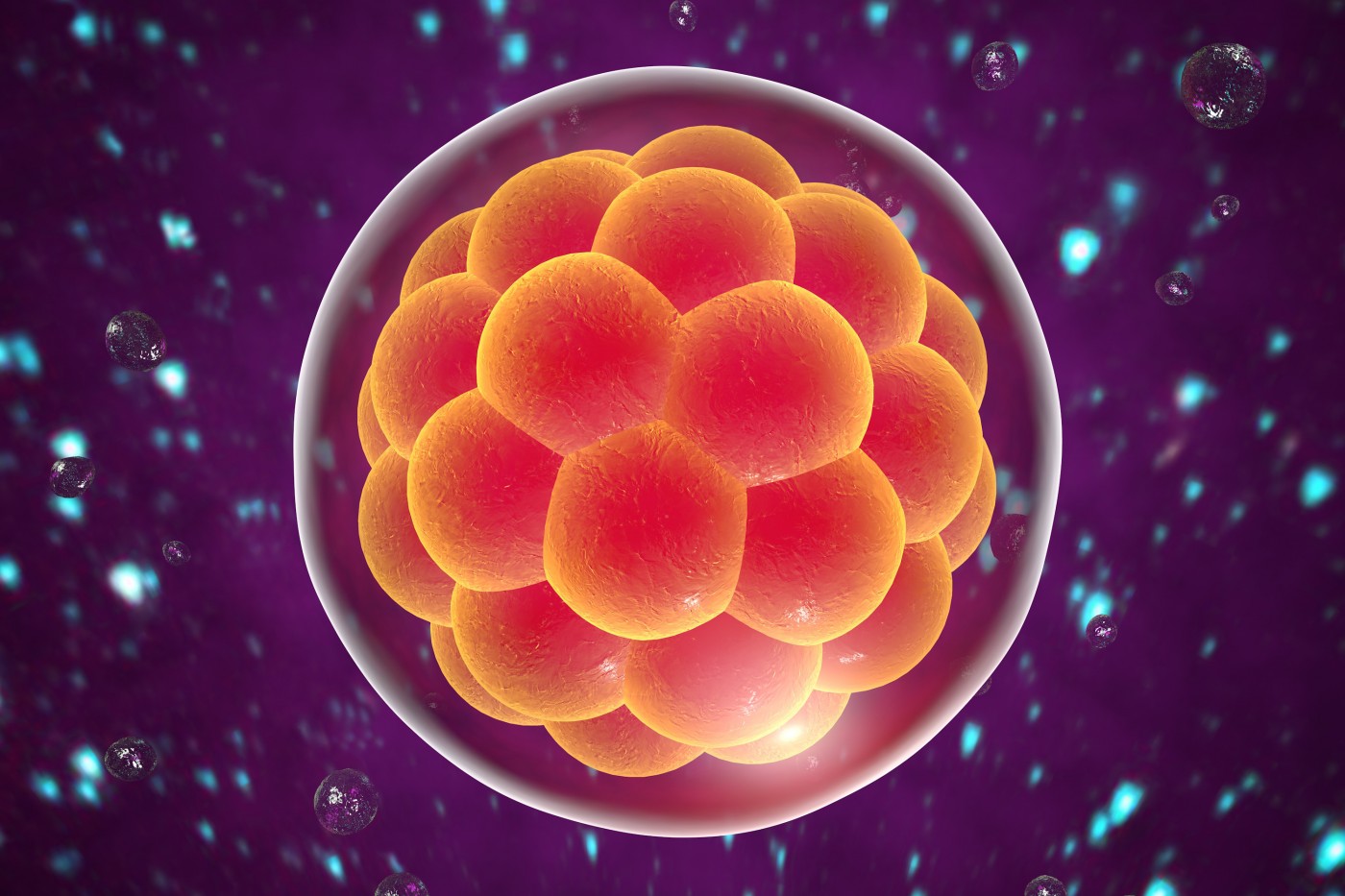
New knowledge in stem cell research may help scientists advance cell therapies for motor neuron diseases such as spinal muscular atrophy (SMA) and amyotrophic lateral sclerosis (ALS).
Human neurons are as diverse as other cell types. Understanding how neurons diversify from stem cells would allow for more precise models of disease and advance drug discovery while leading the way to cell-based neural repair strategies. In a comprehensive overview, University College London researcher Rickie Patani explains how stem cells develop into regions and subtype-specific neurons, and how that knowledge may help researchers advance cell therapies for motor neuron diseases such as spinal muscular atrophy (SMA).
The review, “Generating Diverse Spinal Motor Neuron Subtypes from Human Pluripotent Stem Cells,“ was published in the journal Stem Cells International.
A host of signals applied in sequences, layers and combinations are needed for a cell to reach its destined identity during the process of embryo development. All neurons stem from what is known as the neural tube – a tube of stem cells running from the part of the body where the head will eventually form into the parts destined to become the lower spine. Spinal neurons mature based on graded levels of fibroblast growth factor and retinoic acid exerting their effects via induction of an array of Hox genes (Hox 1-10). The exact concentration of the factors and type of Hox gene is designed to send cells to a specific type of spinal cord neuron along the “head-to-toe” axis.
Other signals determine where, on the “back-to-front” axis, neurons will end up. A signaling factor called Sonic Hedgehog – named after a video game character popular in the 1980s – is crucial for the development of motor neurons along this axis. Researchers know that Sonic Hedgehog signaling occurs in two phases, where varying concentrations of the factor induces stem cells to develop into motor neurons or interneurons that connect different neurons to each other. In addition, researchers believe that Sonic Hedgehog triggers various combinations of transcription factor signaling allowing for the further differentiation of motor neurons into three distinct subtypes.
Motor neuron subclasses are developed along so-called motor columns – discrete columns traveling the length of the neural tube. Each column contains cells destined to become neurons serving postural functions, neurons supporting the respiration or the limb muscles and neurons connecting to the sympathetic ganglia in the spinal cord – relay stations for spinal cord neurons. These columns show different profiles of their gene expression and are further specified by retinoic acid signaling. Moreover, Hox genes again come into play to further drive the development of specific neuronal types.
Since Hox expression seems relatively unspecific, researchers believe that additional regulatory factors are needed to specify cell fate. The transcription factor foxp1 is thought to play an important part in this additional signaling.
Stem cells are cells that have not yet been exposed to the array of signaling events driving their specification. Embryonic stem cells have the biggest potential for turning into a variety of different cells in the laboratory. These cells are so-called pluripotent stem cells with the unique properties to self-renew, develop into any kind of cell in the body, and form genetic chimeras – cells harboring the mix of DNA from two individuals. Human pluripotent stem cells are isolated from 4- to 5-day-old embryos before the embryo is implanted in the uterus. The embryos for human stem cell acquisition are usually donated when there is a surplus of eggs after in vitro fertilization by individuals who sign consent statements.
Stem cells isolated from later stages are already specialized to a certain extent. These multipotent stem cells have the ability to become some pre-defined cells, dependent on the site of isolation. Pluripotent stem cells are therefore the ideal candidates for cell therapy or research work, but the ethical controversies surrounding the use of human embryos have hampered the evolution of this line of research.
The opposition to the human embryonic stem cell work, however, drove the expansion of alternative techniques. Today, inducible pluripotent stem cells can be derived from adult cells by forcing the cells to backtrack their development using a restricted number of signaling factors.
Studies exploring forward programming and transdifferentiation of one differentiated cell type into another has been reporting successful results where skin fibroblasts have been developed into functional and regionally defined neurons. By using the knowledge from studies of embryonal neuronal development, production of functional motor neurons from adult stem cells can be achieved, and has been employed in animal models of spinal cord injury and spinal neuron disorders such as SMA. Still, many questions remain to be answered to optimize stem cell therapies in humans.
Analyses of the timing of neural patterning before final differentiation of cells as well as the exploration of sequential versus simultaneous administration of developmental signals need to be addressed. Such studies will aid in the understanding of mechanisms underlying the generation of neuronal diversity by mimicking in vivo — in living — motor neuron differentiation processes.
Today, motor neuron induction from either embryonic or induced pluripotent stem cells is a rather crude process, and the molecular mechanisms responsible for cellular changes in non-diving cells driving the differentiation of motor neuron subtypes are poorly understood. Experimentation with the application of developmental signals and various culture conditions is used to introduce a larger variation of motor neuron types which researchers are able to produce in the lab.
The author argues that the key to future success of creating motor neuron diversity lies in controlling the instructions during both precursor specification and final differentiation stages of motor neuron development. Moreover, Patani said, that given the profound differences in neuronal architecture, computational power, and functional capacity between rodent and human neurons, studies of human cells are crucial in complementing the knowledge we can gain from animal experiments.