With Zolgensma, Gene Therapy Takes Big Step Forward in Treating Rare Diseases
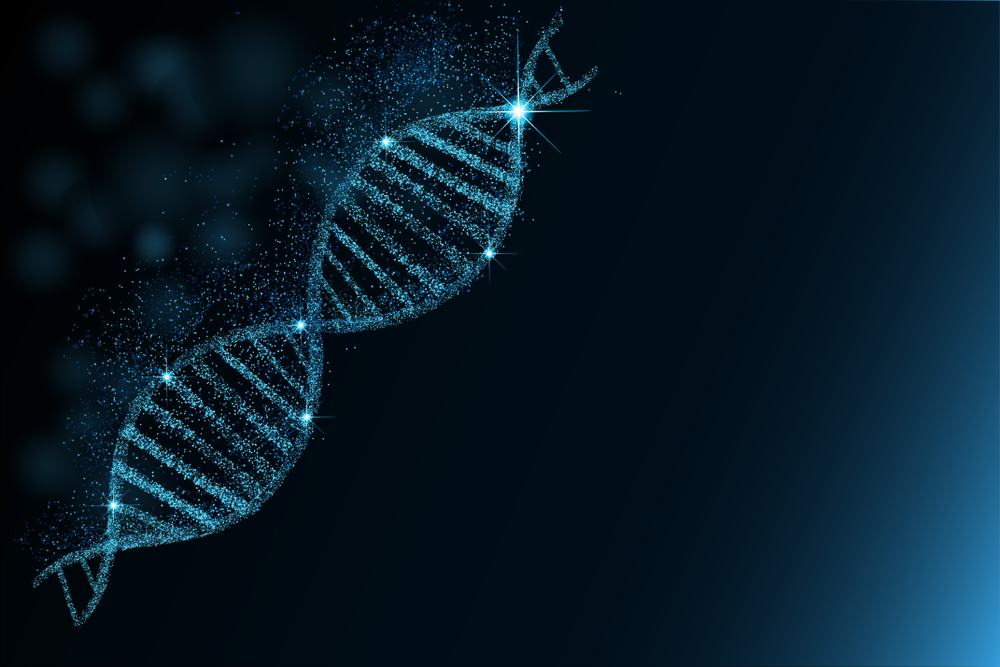
Zolgensma‘s approval as the first gene therapy for treating all forms of spinal muscular atrophy (SMA) in children up to 2 years old is another step forward — an exceptional and far-reaching one — in the seeming revolution underway in treating more diseases, and an increasing number of rare ones, by correcting problems in the illness-causing gene itself.
Long out of reach for many with genetic disorders, gene therapy was first studied in cancer clinical trials dating back to the late 1980s. In the past few years, a number of such therapies have been approved for certain cancers, such as Kymriah by Novartis, and for retinal disease, like Luxturna by Spark Therapeutics, with many more in clinical trials.
Zolgensma (onasemnogene abeparvovec-xioi) is the first gene therapy approved for a chronic neurologic disease.
Genetic mutations can cause the body to stop producing, or produce too few important proteins, or to build deformed proteins — all of which can interfere with normal function and cause disease. Many existing treatments work to mitigate the symptoms these mutations cause. Some go so far as to try to replace missing proteins or remove damaged ones. But very few are able to actually change the genes to enable the body to produce the correct proteins at the proper levels from the outset.
The term “gene therapy” refers to any type of treatment that adds, removes, or changes a patient’s genetic material. Its goal is to treat the underlying cause of a genetic disease rather than its symptoms.
The idea of gene therapy has been around for about 40 years, and researchers regularly use it in the lab to create and study animal models of disease. But it wasn’t until recently that scientists began to find safe and reliable ways of administering it to humans.
Its first U.S. use was in two children with adenosine deaminase (ADA) deficiency, an inherited disease that damages the immune system and leaves people defenseless against infections. The procedure, done at the National Institutes of Health in September 1990, involved removing and “correcting” the genetic material of specific white blood cells, which were then returned to the patients’ bodies. Both children recovered and are “leading normal lives,” the NIH reports.
But obstacles remain.
When it comes to treating people, the main difficulty is delivery. Scientists can’t just identify a faulty gene, go into a patient’s body, then cut up and replace genes one by one. They need to find a way to get as many functional genes as possible into the cells that need them at one go — and without altering genes in other cells.
Michele Calos, PhD, a Stanford professor and president of the American Society for Gene and Cell Therapy, told SMA News Today that the field of gene therapy is now seeing “very rapid growth … And that’s because we’ve spent 20, 30 years figuring out how to do these things.”
Animal models
Researchers manipulate genes in animal models of disease to study what they do and affect. One common strategy is to create a knockout — an animal model in which a particular gene is silenced, or “knocked out,” so that it no longer functions — to understand that gene’s role in the animal’s health and behavior.
Justin Lathia, PhD, who studies an aggressive type of brain cancer called glioblastoma using animal models at the Cleveland Clinic in Ohio, told SMA News Today he considers it “really challenging these days to not have a research project involve a genetic manipulation of some sort.”
The most typical approach used to manipulate genes in both animals and people is through viral vectors.
Viral vectors
Scientists can’t cut open cells and replace their DNA, even if those cells are taken outside a living being to be manipulated. And they can’t inject DNA into the bloodstream, expect it to find its way into the nucleus of only damaged cells, and make the cells start working properly.
“If you just inject DNA into the bloodstream, it gets degraded very quickly before it can actually deliver to any tissues,” Calos said. “In general, it works better if you coat the DNA with, for example, a viral capsid.”
A variety of different methods are now in use for altering cells in animals, usually containing some type of vector — or transfer agent — that can carry desired DNA or RNA and introduce it into a cell’s nucleus.
Viruses — which are smaller than bacteria and consist of only a piece of DNA or RNA that’s packaged inside a molecular envelope — are naturally able to infect cells, typically making people sick. When a virus enters a cell, it inserts its own genetic material into the DNA of that cell, which is then tricked into using its own machinery to replicate the viral DNA and produce more viruses.
Decades ago, scientists managed to harness the virus’ ability to infiltrate cells and insert its own DNA. They start by removing the genetic material that causes infections, rendering it harmless. Then, they add the DNA or RNA capable of altering the expression of a target gene, allowing it to potentially produce things like proteins in beneficial amounts or ways.
Using this “viral vector” approach, researchers can create animal models of diseases — or cells and molecules in a lab dish — that light up and readily show what is happening, where and how, under a microscope. This type of approach was used to develop the therapy that became Zolgensma, scientists at AveXis (now part of Novartis) said in a February 2018 interview.
Of course, this is not a simple process. Since it’s difficult to control where viruses will go in the body, researchers must find ways to ensure they will target and invade the specific cells needing the genetic material it’s carrying, and do so in the correct part of the body.
Genes function differently in different tissues, so if a DNA-carrying virus infects a wrong area, it can do damage or cause unintended outcomes.
“It’s the single most difficult aspect of gene therapy,” Calos said. “How you get this DNA that we can make in a lab … into the correct cells in the body, and get it to stay there also permanently and keep expressing the genes.”
Moreover, researchers have to get the virus to infect enough cells within the tissue to be able to “correct” the problem, but not so many that they trigger an immune response.
It’s a misconception that the virus will infect every cell, Lathias said, noting “the infection rate is not as high as people think it is.”
These difficulties — especially the potential for missing targets or triggering unwanted responses — account for why few gene therapies are in use. However, as knowledge advances, and “transformative” therapies like Zolgensma are developed, that is changing. Great strides already are evident.
“From the basic biology standpoint,” Lathias said, “the gene therapy technology has revolutionized our understanding of the underlying fundamental biology.”
Ex-vivo gene and cell therapies
Before gene therapy, there was cell therapy — basically, replacing a patient’s cells to treat a disease. Blood transfusions, bone marrow transplants, and stem cell therapies are all forms of cell therapy.
Some of the earliest successes with gene therapy overlap with cell therapy, using a strategy called ex-vivo (outside the body) gene therapy. The affected cells are removed from a patient and cultured in petri dishes. Scientists alter the cells’ genetic material and then transplant the newly-functional DNA back into the patient.
CAR-T therapy, under development for certain types of cancer, is an example of this approach. Researchers remove T-cells — immune cells that travel through the blood — and engineer them in the laboratory to express a receptor that helps them recognize and attack cancer cells. The engineered T-cells are injected into the patient to better fight the cancer.
The first ex-vivo therapy, Novartis’ Kymriah, was approved by the U.S. Food and Drug Administration (FDA) in August 2017 to treat acute lymphoblastic leukemia. A few months later, in October 2017, Yescarta by Kite Pharma was approved for some types of large B-Cell lymphoma.
“Gene therapy in blood diseases has been one of the most successful so far because we could use that ex vivo strategy,” Calos sid.
In-vivo gene therapy
But not all cells can be removed from a patient and then reintroduced once a scientist has corrected them. Diseased tissues are often hard to reach.
Scientists must ensure the gene fits in the viral vector, so it can be directed to the tissue it needs to infiltrate. They also must make sure the vector inserts the genetic material into enough cells to improve the patient’s health.
The first such therapy to earn FDA approval for treating a genetic disease was Luxturna, in December 2017. The therapy treats retinal dystrophy, a rare disease that leads to blindness.
The eye was a “smart choice” for one of the first gene therapies, Calos said, because the therapy stays in a small place — one that is “relatively immune deficient.”
From there, investigators and clinicians worked on ways to deliver functional genes to harder-to-reach cells, like motor neurons, which control movement. Patients with SMA lack a functional SMN1 gene, which makes the needed SMN (survival motor neuron) protein.
Zolgensma contains a functional SMN1 gene packaged in an adeno-associated virus (AAV), which is delivered intravenously (in the vein), usually with a single injection.
AAV is “one of the most efficient [vectors] at delivering genes in the body,” Calos said. That assessment is backed by data from Zolgensma’s pivotal Phase 1 clinical trial (NCT02122952) in 15 babies, enrolled before age 6 months, with the most severe form of SMA.
Gene therapies for other diseases, including Duchenne muscular dystrophy (DMD), are entering clinical trials after more than a decade of research. Clinical trials also are ongoing for sickle cell disease and hemophilia, while those for other single-gene diseases like cystic fibrosis are advancing.
Other approaches
While viral vectors are the most common tool scientists are using to deliver gene therapies, several others are in development. One — CRISPR-Cas9 — has taken off during the past half-decade, after scientists discovered that a known function of certain bacteria could be harnessed to cut out and, in some cases, replace individual genes.
The CRISPR-Cas9 system is an adaptation of the natural gene editing method used by bacteria to protect against viruses. Bacteria cut snippets of DNA from a virus to save, and “remember” for future attacks, while also disabling the virus.
Scientists have used the technology in the lab since 2014 to alter genes in a variety of organisms. The system is cheaper, faster, and generally more precise than viral vectors.
It works by cutting out part of a strand of DNA and then allowing the cell to stitch that strand back together, either without the removed segment or with a new gene in its place.
While not quite as precise as hoped, researchers are forging ahead with CRISPR-Cas9 work on cells and animal models, with the goal of making gene therapies accessible to as many patients as possible with genetic disorders.
“The concepts for gene therapy were around in the 1980s,” Calos said. “But it really took a good 20 years before that basic idea was working — not because it wasn’t valid, but just getting each of those steps in the process efficient enough that you could have an impact on the disease.”
More information
The American Society of Gene & Cell Therapy has produced several videos on SMA and gene and cell therapy that may be viewed on its website. With nearly 2,500 members in the U.S. and worldwide, the society is the largest association of individuals involved in gene and cell therapy research.
What is SMA?
What is gene therapy?
Difference between gene and cell therapy: